Science provides evidences for how crooked humans have viewed sexuality
- XX XY
- Dec 20, 2018
- 5 min read
Updated: Jul 8, 2023
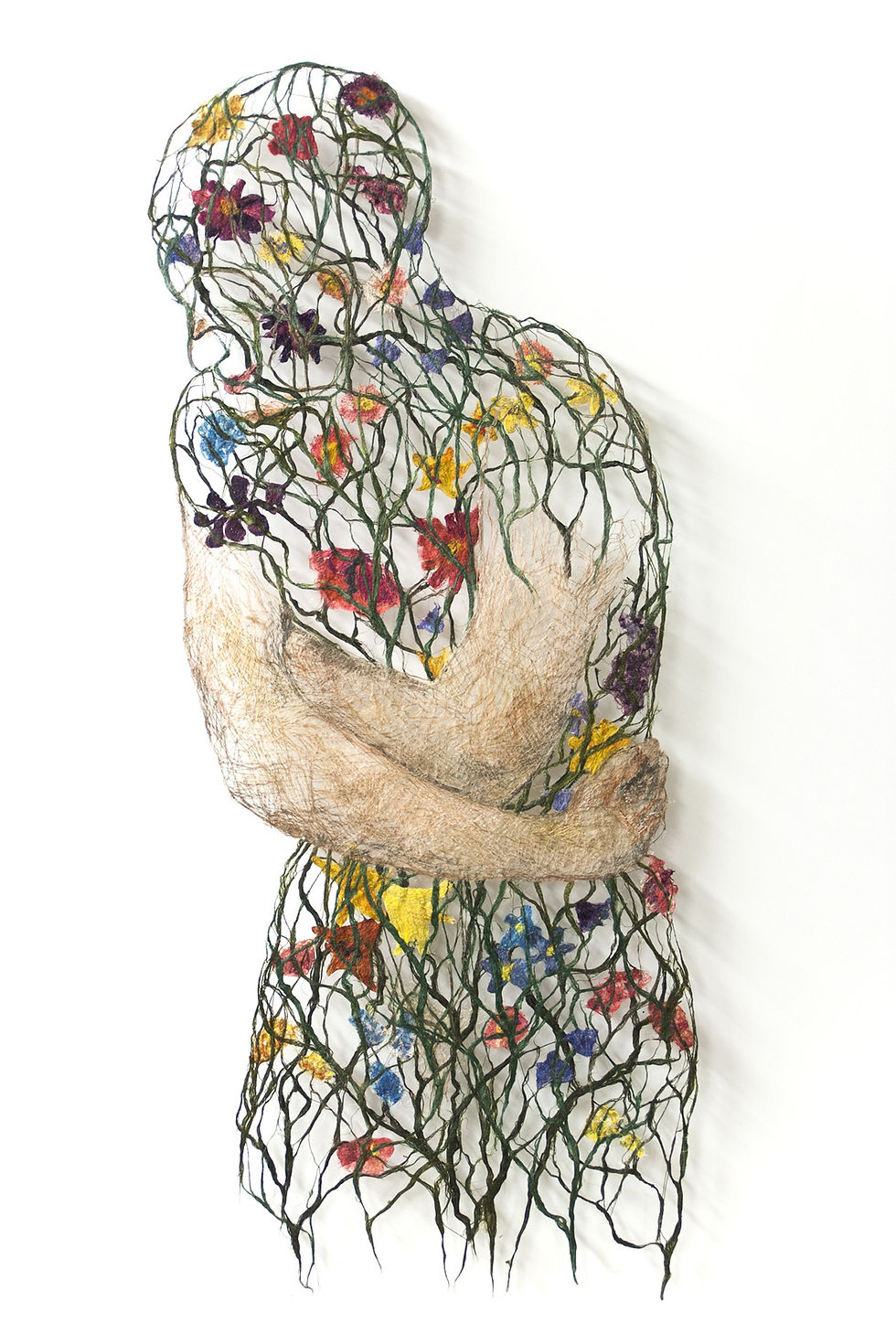
Biologically speaking, men and women belong to the same species and share a common evolutionary ancestor. However, their reproductive function has become the defining characteristic that sets them apart, with the exception of hermaphrodites. Understanding the division of species into two sexes is crucial in comprehending human sexuality.
In a hypothetical sense, all living creatures, including humans, originated from a universal ancestor that exhibited simple morphological traits similar to prokaryotes [1-9]. This ancestral evidence demonstrates that the division of species into two sexes was once unnecessary. Unlike us, our ancestors primarily reproduced asexually through a process called binary fission. This involved the "mother cell" dividing into two identical daughter cells, each possessing the same DNA as the parent cell. Sexual reproduction and meiosis likely evolved simultaneously. Wilkins and Holliday [10] proposed that diploid cells arose when early haploid eukaryotic ancestors started merging with neighboring haploid cells. The transition from haploid to diploid existence, as well as the shift from asexual to sexual reproduction, took millions of years. During this time, various cryptic organisms emerged, marking a transitional stage in the evolutionary tree of life.
Parthenogenesis, a natural mode of asexual reproduction in animals, enables the growth and development of unfertilized embryos [11]. This type of reproduction can be observed in different plant and animal species [12-17]. For instance, crop pests like aphids [12], human parasites such as trematodes [13], and Daphnia [14] undergo cycles of asexual reproduction followed by sexual reproduction in their life cycles. This is referred to as cyclical parthenogenesis. Many plant species can also switch from predominantly asexual reproduction to sexual reproduction when they face harsh or stressful environmental conditions [15]. As a coping mechanism under stress, sexual reproduction allows their genes to combine with new gene sets, potentially enhancing their phenotypes and helping them withstand the stressors. Female North American cottonmouth and copperhead pitvipers [16], as well as the Komodo dragon [17], are capable of reproducing without sex when environmental conditions necessitate it, such as female isolation. In these cases, females can reproduce without the need for sperm.
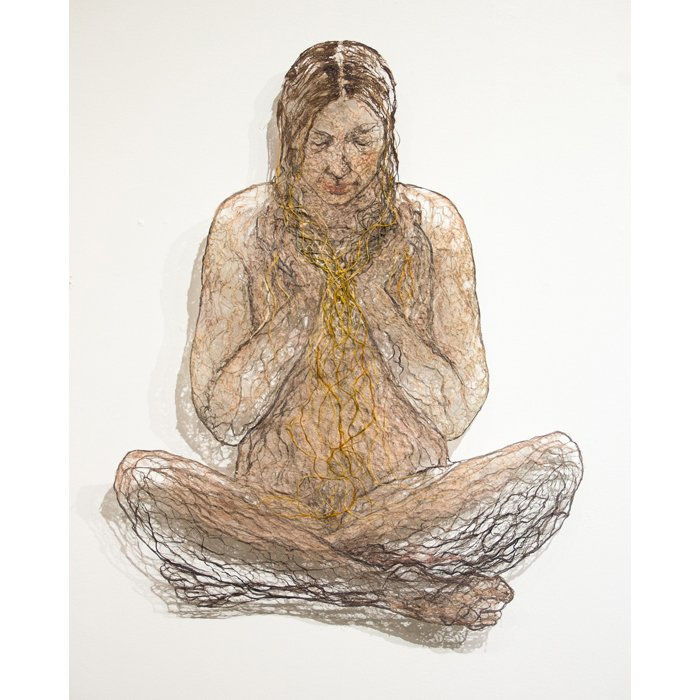
Throughout evolution, various factors such as variation, inheritance, and natural selection have shaped and allowed sexually reproducing organisms to thrive on Earth. Even before Charles Darwin's understanding of genes and evolution, he proposed the concept of natural selection, wherein the fittest organisms are favored [18]. The strategy of genes related to sex is selfish, aiming to spread and create more copies of themselves to ensure their persistence and success [10].
The human sex chromosomes (X and Y) emerged approximately 200-300 million years ago in early mammals [19-20]. These sex chromosomes evolved from autosomes or non-sex-related chromosomes [19-20]. They originated from animals with separate sexes or those whose sex was determined by environmental cues (e.g., temperature-dependent sex in turtles) [21-22]. They may have also developed from hermaphroditic animals possessing both male and female reproductive organs [23-24]. In humans, distinct male-determining genes can be found within our ancestral autosomes [24]. During the evolution of the Y chromosome, these male-determining genes acquired from one member of the autosomal pair needed to halt the recombination process with its homomorphic proto-sex chromosome (X homolog) to evolve independently [19-20, 24-25]. Recombination suppression required chromosomal rearrangement, specifically inversion of segments from end to end [24-26]. This rearrangement process is known as chromosomal inversion [25-27]. Once inversion occurs, the two distinct sex chromosomes can accumulate mutations independently and differentiate [25-27]. Beneficial mutations for one sex but detrimental for the other are believed to have played a significant role in suppressing recombination between evolving sex chromosomes [25-29].
A tradeoff of recombination suppression in the Y chromosome is that both beneficial and deleterious mutations affect the entire chromosome, whereas mutations on recombining homologous X chromosomes are subject to independent selection pressures [25-29]. Consequently, the Y chromosome has experienced degeneration, retaining only 3% of its original genome [30]. Recent findings suggest that genes other than those involved in sex determination have prevented the Y chromosome from becoming extinct [30-32]. These findings indicate that males are here to stay unless another sex-determining gene evolves.
References
Haldane JBS. Origin of Life. The Rationalist Annual. 1929;148: 3–10
Woese C. The universal ancestor. Proc Natl Acad Sci USA. 1998;95: 6854–6859. pmid:96185
Kearney, M., Fujita, M. K. & Ridenour, J. In Lost sex: the evolutionary biology of parthenogenesis (eds I. Schön, K. Martens, & P. van Dijk) 447–474 (Springer Scientific, 2009).
Watts, PC. et al. Parthenogenesis in Komodo dragons. Nature. 2006; 444, 1021-22
Darwin, Charles. On the Origin of Species by Means of Natural Selection, Or, the Preservation of Favoured Races in the Struggle for Life. London: J. Murray, 1859. Print.
Charlesworth, B. The evolution of sex chromosomes. Science. 1991; 251, 1030–1033
Kirpichnikov VS. Genetic basis of fish selection. New York: Springer-Verlag: 1981
Rice WR. Evolution of the Y sex chromosome in animals. BioScience. 1996;46:331–343.
Ross MT, et al. The DNA sequence of the human X chromosome. Nature (London) 2005;434:325–337.
Kirkpatrick M. How and Why Chromosome Inversions Evolve. Plos Biology. 2010;8
Fisher RA. The Genetical Theory of Natural Selection. Oxford University Press; Oxford: 1930
Comments